Nanocomposite Tectons
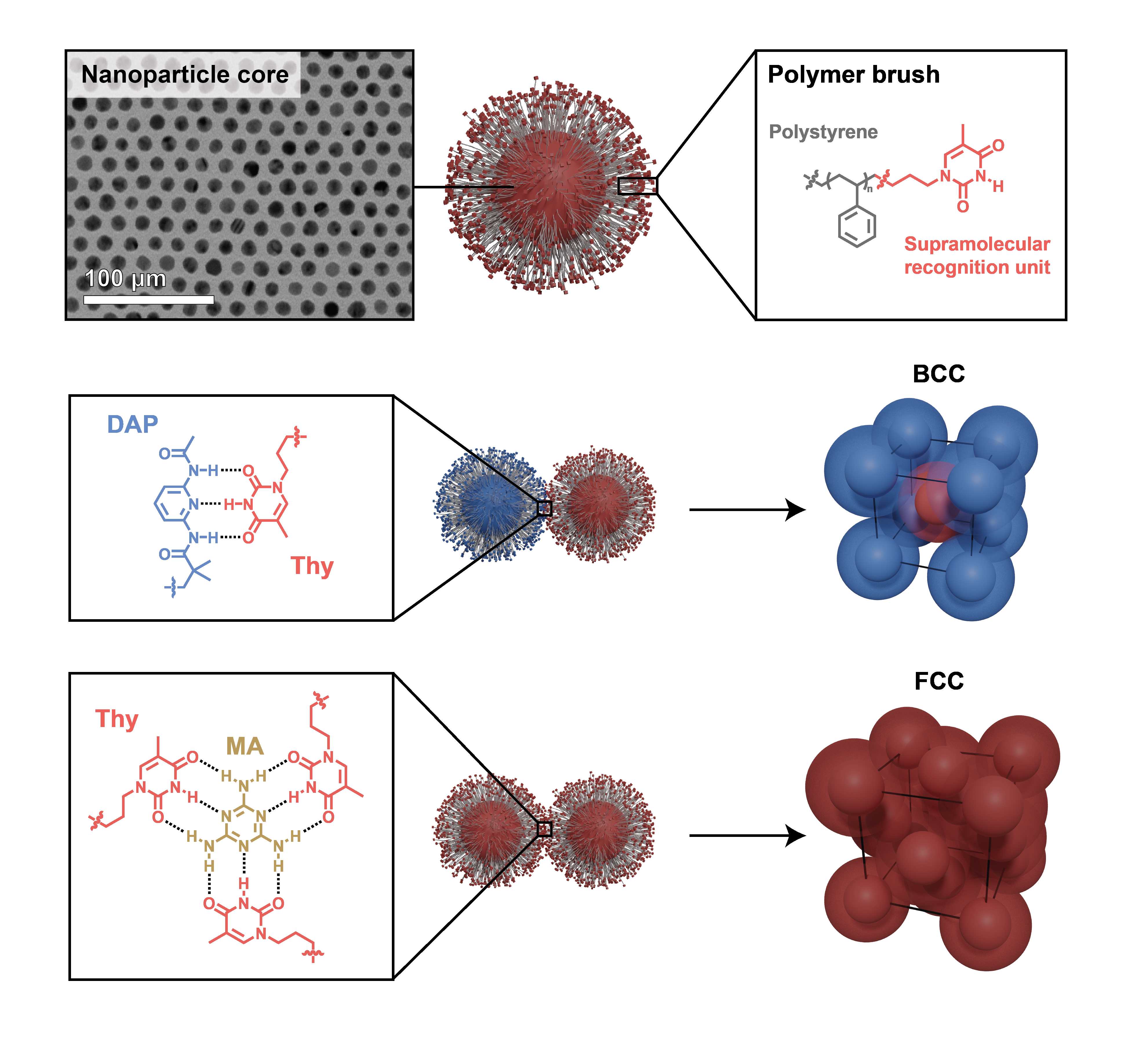
The Macfarlane lab is focused on the self-assembly of nanoscopic components as a means of tuning the bulk
properties of composite materials. In order to achieve this goal, we are broadly interested in the research
areas of soft matter, self-assembly, nanoparticle synthesis, polymer chemistry, and biomaterials.
The nanoscale building blocks we use range from inorganic nanoparticles to synthetic polymers to biomolecules
like DNA, and the materials we aim to develop possess interesting optical, chemical, electrical, and mechanical
properties. The emergent properties of these structures will have significant impact in energy research via
light manipulation (e.g. photonic band gaps or plasmonic metamaterials), electronic device fabrication (e.g.
semiconducting substrates or data storage devices), and medical research (e.g. hydrogels for
sustained drug delivery).
Organizing matter with precision to achieve control over the properties and functions of nanomaterials
Blending diverse materials to create enhanced, multifunctional composites with tailored properties and applications
Fabricating, shaping, and refining materials to optimize properties and enable production at scale
Engineering of materials and devices at the nanoscale, enabling novel properties and applications
Nanocomposites enable the integration of nanoscale phenomena into functional, macroscopic materials by carefully controlling the composition and spatial configuration of the constituent components. Self-assembly of smaller building blocks (tectons) is a powerful method for synthesizing nanocomposites because it can precisely dictate nanoscale structure in a scalable manner. Our group has developed a set of building blocks, Nanocomposite Tectons (NCTs), which are themselves organic/inorganic nanocomposites capable of self-assembling into larger, ordered structures. Each NCT consists of an inorganic nanoparticle grafted with a dense layer of polymer chains that terminate in molecular recognition units capable of programmed supramolecular bonding.
The modular nature of NCTs provides multiple design handles to alter the composition, size, and thermodynamics of assembly to introduce new geometric arrangements and properties of the resulting material. As a result, these structures have potential application in the areas of plasmonics and photonics, heterogeneous catalysis, and energy storage.
Adding inorganic filler components into a polymer matrix is a common method of creating high-performance polymer-inorganic nanocomposites. These materials can possess ideal mechanical properties and ease of processability via a continuous polymer network while maintaining the functionalities of brittle inorganic fillers. To improve these materials, it is critical to develop a technique to embed large quantities of inorganic fillers into a polymer matrix without aggregation, as to not to sacrifice mechanical performance. Polymer-grafted nanoparticles (PGNPs) are single-component building blocks for nanocomposites with regularly distributed, large quantities of inorganic filler. Specifically, our group has developed crosslinkable nanoparticles (XNPs) by employing versatile chemical strategies that crosslink polymer networks, including additives, thermal aging, and ionic interactions. XNPs enable transformable, mechanically robust nanocomposites containing over 30 vol% of homogeneously distributed fillers at a macroscopic scale. PGNP and XNP concepts can be expanded to combinations of various polymers and inorganic filler materials, and investigated for applications such as thermal, electrical, and photonic materials.
DNA-coated nanoparticles are a powerful synthon for materials development, as nanoparticles possess size, shape, and composition dependent physical properties, and the sequence dependent recognition properties of DNA allow one to precisely organize particles into well-defined crystalline lattices with nanometer-scale precision. The ability to generate lattices with precise control over both the identity of the particles as well as their positions in three-dimensions has implications for developing materials for applications in areas ranging from photonics to catalysis to energy generation and storage.
In this research area, we utilize both DNA-based particle assembly techniques and top-down lithographic methodologies to explore fundamental concepts of materials science (such as thermodynamics and kinetics of epitaxial deposition, thickness-induced melting suppression, lattice strain, defect structure, dewetting, etc.) using these nanoscale “atom equivalents”. We also utilize these building blocks to generate materials where we can precisely study structure-property relationships (such as their mechanical property, asymmetric reflectivity, shape-induced plasmonic response, etc.) in structures that have nanometer scale ordering, leading towards practical applications such as micro-mirrors in ultra-flat optical devices.